The role of a bioreactor is to provide the ideal environment for cells to focus on what they are supposed to do. With many configurations available on the market, it is often a struggle to choose which system is best suited for your operation. Initially, when selecting a bioreactor, you can choose stainless steel (SS) or single-use (SU) technologies. We discuss the pros and cons of SS and SU in our Modern Laboratory blog.
When it comes to SU, alongside the Cellexus CellMaker airlift bioreactor, there are 2 key bioreactor configurations on the market:
1. Pneumatic Airlift
Mixing entirely driven by purged air through submerged sparger. Oxygen transfer occurs via rising bubbles and headspace. No internal moving parts.
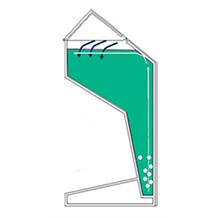
Figure 1. Pneumatic Airlift.
2. Oscillating Wave Bag
Mixing entirely driven by ‘wave’ motion of reactor. Oxygen transfer driven by headspace. No internal moving parts or submerged sparging.
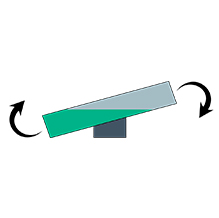
Figure 2. Oscillating Wave Bag.
3. Stirred Tank
Mixing driven by internal impeller (may also include baffles). Aeration driven by submerged sparger located under impeller.
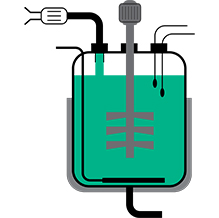
Figure 3. Stirred tank.
Oscillating Wave Bag
Launched in the mid-90’s, the wave rocking bioreactor was the first SU bioreactor design. Analogous to the CellMaker it features no internal moving parts. Its gentle agitation is driven by swaying-motion of the reactor bag on a rocking tray. This results in low shear stress – highly suitable for fragile mammalian cell lines. The design of these bags, however, has inherent limitations. The scale-up capacity is affected by the design of the bag, which can only handle working volumes at half of the actual bag volume. No sparged gas restricts the gas exchange rations, thus these bags are suitable only for cultivation of mammalian cells. The bacterial and yeast would struggle to achieve high cell densities in this type of a bioreactor due to their high oxygen demand.
Single-use Stirred Tank
Perhaps due to their popularity in the reusable reactor space, stirred tank reactor designs are also the most popular design when it comes to SU systems. These systems are known for their ability to deliver extremely high cell culture densities, but at the cost of equally high shear stress to the cells. This causes complications when growing shear-sensitive cell types in a stirred tank bioreactor and also not all bioproducts will remain stable in such conditions. Mirroring several design developments made in their SS predecessors, a lot of these essential features do not cross over as well to the SU. In particular, a stirred tank bioreactor requires an impeller to mix the liquor which inherently complicates the SU design as a cumbersome motor needs to be implemented (further risking sterility). Other issues with the CSTR designs are the inefficient methods of temperature control e.g., awkward heating jackets. The more complicated a system gets, the total of price additional materials (metals for impellers, spargers, internal brackets, seals) starts to compound. Furthermore, the type of propellor chosen forces a compromise between shear and mixing efficiency.
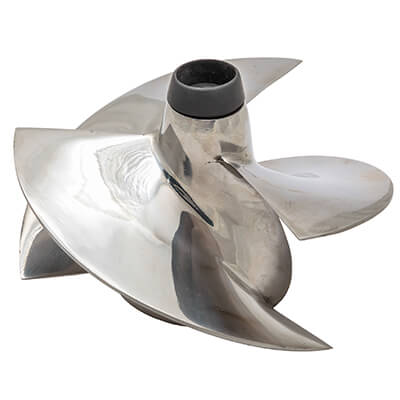
Figure 4. Marine-styled impellers allow more gentle mixing but at reduced mixing efficiency.
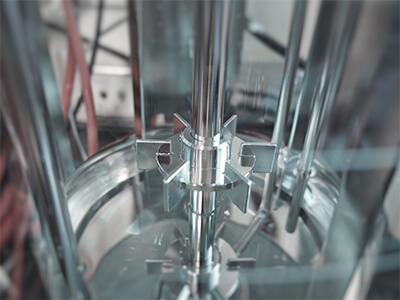
Figure 5. Rushton impellers are more aggressive in their ability to produce turbulent, well-mixed flow however are not suitable for sensitive cell lines as this mixing is coupled with high mechanical shear.
Pneumatic Airlift
Airlift technology is not a new technology, but it has been absent in bioprocessing until relatively recently. Unlike classic pneumatically agitated airlift reactors where liquid mixing is random (bubble column), the CellMaker’s patented bioreactor geometry means that liquid mixing flow is designed to circulate (driven by the gas sparger). In airlift bioreactors, liquid circulation between the riser and downcomer is the main contributor to fluid dynamics. This contrasts with stirred tank reactors where localized impeller mixing generates large shear gradients that cause cells to experience mechanical stress, as well as stress in areas of varied solutes concentrations (O2, CO2, H+, metabolites) and temperature imbalances.
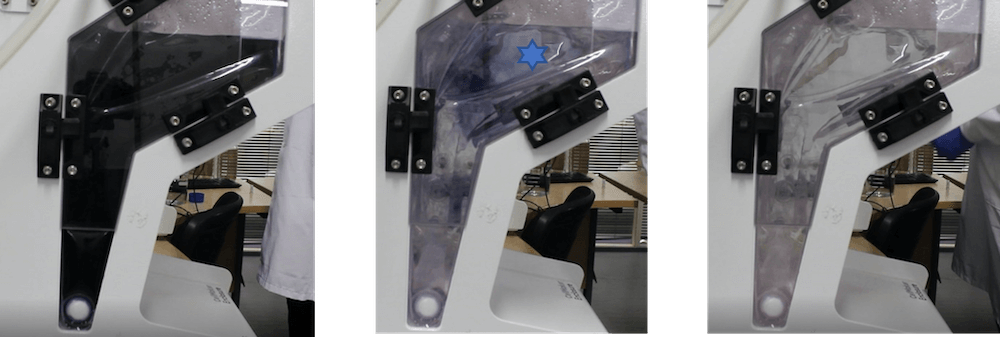
Figure 6. Iodometry Decolourisation Mixing Assay
Figure 6 shows there is no intense focal point of energy dissipation in the bioreactor. With our unique geometry, the liquid is gently vortexed in the upper section (blue star) before settling. In other bioreactors, it is common to experience mixing dead zones at the farthest point to the mixing driver (impeller); baffles are implemented to combat this effect by disrupting distal laminar flow. As seen in Figure 6 the last zone of incomplete mixing in the CellMaker is the center of the reactor which confirms homogeneous mixing and energy (shear) distribution. We believe these lower cellular shear forces are at the core of our universal success across multiple cell lines. Furthermore, with cell media occupying a significant share of process costs, Cellexus has found that the simplicity of our SU bag design means there is less likelihood for operational error, mechanical failure and – very important to us – risk of contamination.
Conclusion
In short, there are many routes to be taken when choosing upstream configurations. When it comes to SU bioreactors it is important to address both the biology of your system as well as the logistics of your manufacturing scale up. At Cellexus we have successfully maintained simplicity of use without sacrificing performance metrics.